Researchers at the University of Tennessee have successfully developed a key technology related to an experimental fusion reactor.
The critical step achieved by ITER – a project involving the United States, five other nations and the European Union? Insulating and stabilizing the central solenoid, otherwise known as the reactor’s backbone.
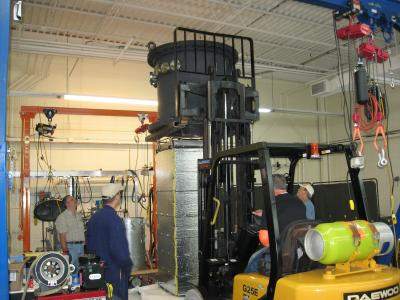
Indeed, ITER is building a fusion reactor that aims to produce 10 times the amount of energy that it uses. The facility is currently under construction near Cadarache, France and is slated to begin operations in 2020.
“The goal of ITER is to help bring fusion power to the commercial market. Fusion power is safer and more efficient than nuclear fission power,” explained Professor Madhu Madhukar.
“There is no danger of runaway reactions like what happened in nuclear fission reactions in Japan and Chernobyl, and there is little radioactive waste. Unlike today’s nuclear fission reactors, fusion uses a similar process as that which powers the sun.”
Since 2008, UT engineering professors and about 15 students have worked inside the Magnet Development Laboratory (MDL) to develop technology that serves to insulate and provide structural integrity to the more than 1,000 ton central solenoid.
A tokamak reactor uses magnetic fields to confine the plasma – a hot, electrically charged gas that serves as the reactor fuel – into the shape of a torus. The central solenoid, which consists of six giant coils stacked on top of one another, plays the primary role by both igniting and steering the plasma current.
The key to unlocking the technology was finding the right material – a glass fiber and epoxy chemical mixture that is liquid at high temperatures and turns hard when cured – and the correct process of inserting this material into all of the necessary spaces inside the central solenoid.
The special mixture provides both electrical insulation and strength to the heavy structure. The impregnation process moves the material at the right pace, factoring in a range of variables, including temperature, pressure, vacuum and the material’s flow rate.
“During the epoxy impregnation, we were in a race against time,” said Madhukar. “With the epoxy, we have these competing parameters. The higher the temperature, the lower the viscosity; but at the same time, the higher the temperature, the shorter the working life of the epoxy.”
It took two years to develop the technology, more than two days to impregnate the central solenoid mockup and multiple pairs of watchful eyes to ensure everything went according to plan.
The next step? The team’s technology will be transferred to US ITER industry partner General Atomics in San Diego, which will build the central solenoid and subsequently ship it to France.