Radiation causes ten times as much structural damage to electronic materials at the atomic level as previously believed, say scientists using a new investigation technique.
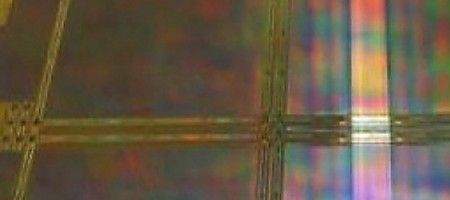
The Vanderbilt University team used a combination of lasers and acoustic waves to pinpoint the size and location of defects buried deep inside with greater precision than ever before.
“The ability to accurately measure the defects in electronic materials becomes increasingly important as the size of microelectronic devices continues to shrink,” says physics professor Norman Tolk.
“When an individual transistor contains millions of atoms, it can absorb quite a bit of damage before it fails. But when a transistor contains a few thousand atoms, a single defect can cause it to stop working.”
Previous methods used to study damage in electronic materials have looked only at defects and deformations in the atomic lattice. The new method, though, can detect disruption in the positions of the electrons that are attached to the atoms – and it’s the behavior of the electrons that determines a material’s electrical and optical properties.
“An analogy is a thousand people floating in a swimming pool. The people represent the atoms and the water represents the electrons,” says post-doctoral fellow Andrew Steigerwald.
“If another person – representing an energetic particle – jumps into the pool, the people in his vicinity change their positions slightly to make room for him. However, these shifts can be fairly subtle and difficult to measure. But the jumper will also cause quite a splash and cause the level of the water in the pool to rise. Much like the water in the pool, the electrons in a material are more sensitive to defects than the atoms.”
To detect the electron dislocations, the physicists upgraded a 15-year-old method called coherent acoustic phonon spectroscopy (CAPS).
“CAPS is similar to the seismic techniques that energy companies use to search for underground oil deposits, only on a much smaller scale,” said Steigerwald.
It generates a pressure wave that passes through a chunk of semiconductor by blasting its surface with an ultrafast pulse of laser light. As this happens, the researchers bounce a second laser off the pressure wave and measure the strength of the reflection.
As the pressure wave encounters defects and deformities in the material, its reflectivity changes and this alters the strength of the reflected laser light. By measuring these variations, the physicists can detect individual defects and measure the effect that they have on the material’s electrical and optical properties.
The physicists tested their technique on a layer of gallium arsenide semiconductor that they had irradiated with high-energy neon atoms. They found that the structural damage caused by an embedded neon atom spread over a volume containing 1,000 atoms – far greater than shown by other techniques.
“This is significant because today people are creating nanodevices that contain thousands of atoms,” says Steigerwald. One of these devices is a solar collector made from quantum dots – and the new results may explain why these are less efficient than predicted.
“The fact is that we really don’t understand how any atomic-scale defect affects the performance on an optoelectronic device,” says Tolk.
“Techniques like the one that we have developed will give us the detailed information we need to figure this out and so help people make nanodevices that work properly.”