Using the world’s most brilliant X-ray source, scientists have for the first time peered into molten magma at conditions of the deep Earth mantle. The analysis at DESY’s light source PETRA III revealed that molten basalt changes its structure when exposed to pressure of up to 60 gigapascals (GPa), corresponding to a depth of about 1400 kilometres below the surface.
At such extreme conditions, the magma changes into a stiffer and denser form, the team around first author Chrystèle Sanloup from the University of Edinburgh reports in the scientific journal Nature. The findings support the concept that the early Earth’s mantle harboured two magma oceans, separated by a crystalline layer. Today, these presumed oceans have crystallised, but molten magma still exists in local patches and maybe thin layers in the mantle.
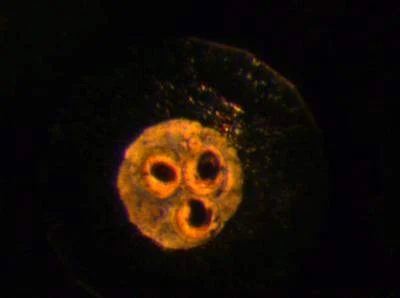
“Silicate liquids like basaltic magma play a key role at all stages of deep Earth evolution, ranging from core and crust formation billions of years ago to volcanic activity today,” Sanloup emphasised. To investigate the behaviour of magma in the deep mantle, the researchers squeezed small pieces of basalt within a diamond anvil cell and applied up to roughly 600,000 times the standard atmospheric pressure. “But to investigate basaltic magma as it still exists in local patches within the Earth’s mantle, we first had to melt the samples,” explained co-author Zuzana Konôpková from DESY, who supported the experiments at the Extreme Conditions Beamline (ECB), P02 at PETRA III.
The team used two strong infrared lasers that each concentrated a power of up to 40 Watts onto an area just 20 micrometres (millionths of a metre) across – that is about 2000 times the power density at the surface of the sun. A clever alignment of the laser optics allowed the team to shoot the heating lasers right through the diamond anvils. With this unique setup, the basalt samples could be heated up to 3,000 degrees Celsius in just a few seconds, until they were completely molten.
To avoid overheating of the diamond anvil cell which would have skewed the X-ray measurements, the heating laser was only switched on for a few seconds before and during the X-ray diffraction patterns were taken. Such short data collection times, crucial for this kind of melting experiments, are only possible thanks to the high X-ray brightness at the ECB. “For the first time, we could study structural changes in molten magma over such a wide range of pressure,” said Konôpková.
The powerful X-rays show that the so-called coordination number of silicon, the most abundant chemical element in magmas, in the melt increases from 4 to 6 under high pressure, meaning that the silicon ions rearrange into a configuration where each has six nearest oxygen neighbours instead of the usual four at ambient conditions. As a result, the basalt density increases from about 2.7 grams per cubic centimetre (g/ccm) at low pressure to almost 5 g/ccm at 60 GPa.
“An important question was how this coordination number change happens in the molten state, and how that affects the physical and chemical properties,” explained Sanloup. “The results show that the coordination number changes from 4 to 6 gradually from 10 GPa to 35 GPa in magmas, and once completed, magmas are much stiffer, that is much less compressible.” In contrast, in mantle silicate crystals, the coordination number change occurs abruptly at 25 GPa, which defines the boundary between the upper and lower mantle.
This behaviour allows for the peculiar possibility of layered magma oceans in the early Earth’s interior.
“At low pressure, magmas are much more compressible than their crystalline counterparts, while they are almost as stiff above 35 GPa,” explained Sanloup. “This implies that early in the history of the Earth, when it started crystallising, magmas may have been negatively buoyant at the bottom of both, upper and lower mantle, resulting in the existence of two magma oceans, separated by a crystalline layer, as has been proposed earlier by other scientists.”
At the high pressure of the lower Earth mantle, the magma becomes so dense that rocks do not sink into it anymore but float on top. This way a crystallised boundary between an upper and a basal magma ocean could have formed within the young Earth. The existence of two separate magma oceans had been postulated to reconcile geochronological estimates for the duration of the magma ocean era with cooling models for molten magma.
While the geochronological estimates yield a duration of a few ten million years for the magma ocean era, cooling models show that a single magma ocean would have cooled much quicker, within just one million years. A crystalline layer would have isolated the lower magma ocean thermally and significantly delayed its cooling down. Today, there are still remnants of the basal magma ocean in the form of melt pockets detected atop the Earth’s core by seismology.