In the baseball world, a superstar can do five things exceptionally well: hit, hit for power, run, throw and field. n the parallel universe of the microbiological world, there is a current superstar species of blue-green algae that, through its powers of photosynthesis and carbon dioxide fixation, or uptake, can produce (count ’em) ethanol, hydrogen, butanol, isobutanol and potentially biodiesel. Now that’s some five-tool player.
In baseball, you call that player Willie Mays or Mike Trout. In microbiology, it goes by Synechocystis 6803, a versatile, specialized bacterium known as a cyanobacterium. It makes pikers out of plants when it comes to capturing and storing energy from photosynthesis, and it’s a natural in converting the greenhouse gas carbon dioxide (CO2) to useful chemicals that could help both tame global warming and sustain energy supply.
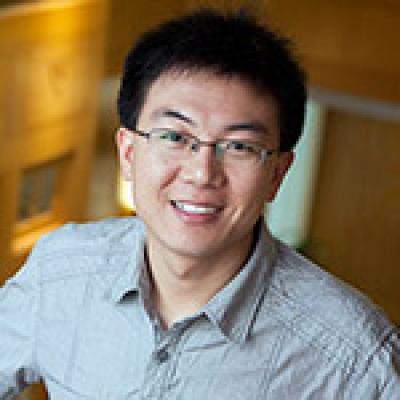
In addition, genetically engineered Synechocystis 6803 also has the potential to make commodity chemicals and pharmaceuticals.
Granted, that’s mostly in laboratories, on the liter scale. Because of its versatility and potential, this microscopic organism is one of the most studied of its kind since it was discovered in 1968. But just as in baseball, where “can’t miss” five-tool prospects are signed yearly with great expectations and never achieve their promise, Synechocystis 6803 has yet to deliver.
Fuzhong Zhang, PhD, assistant professor of energy, environmental & chemical engineering at Washington University in St. Louis, works with Synechocystis 6803 — as well as other microbes and systems — in the areas of synthetic biology, protein engineering and metabolic engineering, with special focus on synthetic control systems to make the organism reach its untapped prowess.
Zhang says the biotech world has to overcome several challenges to put the engineered microbes in the applications stage. Zhang will be in the thick of them.
“My goal is to engineer microbes and turn them into microfactories that produce useful chemicals,” Zhang says. “Synechocystis is particularly interesting because it can use CO2 as the only carbon source. Engineering this bacterium would turn the fixed CO2 into metabolites that can be further converted to fuels and other chemicals through designed biosynthetic pathways.”
Traditional chemical production requires high pressure and temperatures and literally tons of chemical solvents, but the microbial approach is very eco-friendly: Once the engineered cyanobacteria start to grow, all they need are water, basic salts and the CO2.
In an academic “scouting report” of Synechocystis, published in the August 2013 Marine Drugs, Zhang and colleagues summarize recent research and conclude that production speed has to be increased and new genetic tools must be developed to control the biochemistry inside Synechocystis so that chemical productivities will be improved to make this technology economically viable.
Current industry specifications for potentially scalable chemical production are roughly 100 grams per liter of fuel or chemicals. Presently, the laboratory production is generally less than 1 gram per liter, and the efficiency is very low.
Zhang says the research community needs better tools to control gene expression. For example, promoters — little stretches of DNA before genes of interest that help control gene expression — with predictable strength are needed. They also need better cellular biosensors that can sense key metabolites and control the production of vital proteins that create the desired chemicals.
And they need to engineer the organisms’ circadian rhythms (day/night) to someday produce organisms that work around the clock making a fuel or chemical. Natural Synechocystis 6803, for instance, performs a yeoman’s task of producing and storing energy molecules during the day through photosynthesis, but at night, it uses a different set of metabolisms to consume the stored energy.
The natural circadian rhythm has to be rewired to make a biofuel 24 hours a day.
Zhang’s research includes developing gene expression tools, new chemical biosynthetic pathways and circadian control tools for cyanobacteria.
“I’m confident that in two or three years we will have more potent tools to engineer gene expression levels and timing, which will speed up the process more accurately and efficiently,” he says.
Also, his group has been working to develop dynamical control systems in microbes that function like meters and valves in a traditional chemical production plant – the meters calculate pressure and flow, and the valves control them.
“It’s a biological version of the valve-and-meter model to control the flow of metabolites that make the production of fuel and chemicals more efficiently,” he says.