A simple pendulum has two equilibrium points: hanging in the “down” position and perfectly inverted in the “up” position. While the “down” position is a stable equilibrium, the inverted position is definitely not stable. Any infinitesimal deviation from perfectly inverted is enough to cause the pendulum to eventually swing down.
It has been known for more than 100 years, though, that an inverted pendulum can be stabilized by vibrating the pivot point. This non-intuitive phenomenon is known as dynamic stabilization, and it has led to a broad range of applications including charged particle traps, mass spectrometers and high-energy particle accelerators.
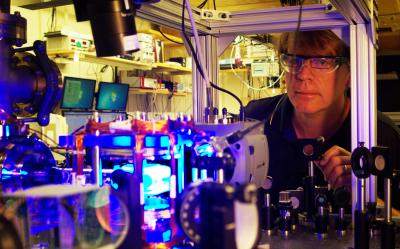
Many-body quantum systems can also be placed into unstable non-equilibrium states, and like the inverted pendulum of classical physics, they typically evolve away from these states. Now, researchers at the Georgia Institute of Technology have demonstrated a way to maintain an unstable quantum system by applying bursts of microwave radiation – a quantum analog to vibrating the inverted pendulum.
In an experiment that could have implications for quantum computers and quantum simulators, the researchers used microwave pulses of varying amplitudes and frequencies to control a quantum system composed of a cloud of approximately 40,000 rubidium atoms cooled nearly to absolute zero.
The research, sponsored by the National Science Foundation and reported online August 27 by the journal Physical Review Letters, experimentally demonstrated dynamical stabilization of a non-equilibrium many-body quantum system. The paper is scheduled to appear in the journal’s August 30 print issue.
“In this work, we have demonstrated that we can control the quantum dynamics of a cloud of atoms to maintain them in a non-equilibrium configuration analogous to the inverted pendulum,” said Michael Chapman, a professor in the Georgia Tech School of Physics. “What we actually control is the internal spins of the atoms that give each atom a small magnetic moment. The spins are oriented in an external magnetic field against their will such that they would prefer to flip their orientation to the equilibrium position.”
Mathematically, the state of the rubidium atoms is virtually identical to that of the simple mechanical pendulum, meaning that Chapman and his students have controlled what could be called a “quantum inverted pendulum.”
In their experiment, the researchers began with a spin-1 atomic Bose-Einstein condensate (BEC) that is initialized in an unstable, fixed point of the spin-nematic phase space – comparable to an inverted pendulum. If allowed to freely evolve, interactions between the atoms would give rise to squeezing, quantum spin mixing and eventually relaxation to a stable state – comparable to a pendulum hanging straight down from a pivot point.
By periodically applying bursts of microwave radiation, the researchers rotated the spin-nematic many-body fluctuations, halting the squeezing and the relaxation toward stability. The researchers investigated a range of pulse periods and phase shifts to map a stability diagram that compares well with what they expected theoretically.
“The net effect is that the many-body system basically returns to the original state,” said Chapman. “We reverse the squeezing of the condensate, and after it again evolves toward squeezing, we cause it to return. If we do this periodically, we can maintain the Bose-Einstein condensate in this unstable point indefinitely.”
The control technique differs from active feedback, which measures the direction in which a system is moving and applies a force counter to that direction. The open-loop technique used by Chapman’s group applies a constant input that doesn’t vary with the activity of the system being controlled.
“We are periodically kicking the system to keep it in a state where it doesn’t want to be,” he said. “This is the first time we have been able to make a many-body spin system that we can stabilize against its natural evolution.”
Controlling and manipulating single-particle quantum systems or simple collections of atoms, electrons and photons has been a focus of the physics community over recent decades. These capabilities have formed the foundation for technologies such as lasers, magnetic resonance imaging, atomic clocks and new atomic sensors for magnetic fields and inertial guidance.
Now, researchers are studying more complex systems that involve many additional interacting particles, perhaps thousands of them. Chapman and his group hope to help extend their knowledge of these more complex many-body systems, which could lead to developments in quantum computing, quantum simulations and improved measurements.
“The long-range goal of our work is to further the understanding of quantum mechanics and to develop new technologies that exploit the often counterintuitive realities of the quantum world,” Chapman said. “Quantum many-body systems are being actively explored, and one of the things you’d like to do is be able to control them. I think this is one of the cleanest examples of being able to control a quantum many-body system in a manifestly unstable configuration.”