A ‘spacetime crystal’ sounds like something Doctor Who might keep in his kit-bag – but it’s actually much more dramatic than that: a clock that could keep perfect time forever, even after the heat-death of the universe.
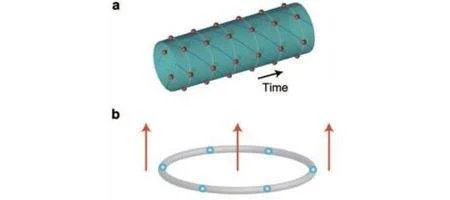
It’s a four-dimensional crystal that has periodic structure in time as well as space, and scientists at Berkeley Lab say they’ve come up with an experimental design for one.
With such a crystal, scientists would be able to study how complex physical properties and behaviors emerge from the collective interactions of large numbers of individual particles, the so-called many-body problem of physics.
It could also be used to study phenomena in the quantum world such as entanglement, in which an action on one particle impacts another particle even if the two are separated by vast distances.
The Berkeley Lab design is based on an electric-field ion trap and the Coulomb repulsion of particles that carry the same electrical charge.
“The electric field of the ion trap holds charged particles in place and Coulomb repulsion causes them to spontaneously form a spatial ring crystal,” says Xiang Zhang, a faculty scientist with Berkeley Lab’s Materials Sciences Division.
“Under the application of a weak static magnetic field, this ring-shaped ion crystal will begin a rotation that will never stop. The persistent rotation of trapped ions produces temporal order, leading to the formation of a space-time crystal at the lowest quantum energy state.”
Because the space-time crystal is already at its lowest quantum energy state, its temporal order, or timekeeping, will theoretically persist even after the rest of our universe reaches thermodynamic equilibrium or ‘heat-death’.
Earlier this year, Frank Wilczek, the Nobel-prize winning MIT physicist, mathematically proved that a time crystal can exist. It’s only now, though, that Zhang and his group have come up with an experimental design.
Crystallization takes place when heat is removed from a molecular system until it reaches its lower energy state. At a certain point, continuous spatial symmetry breaks down and the crystal assumes discrete symmetry, meaning that instead of the structure being the same in all directions, it’s the same in only a few.
And just as a 3D crystal is configured at the lowest quantum energy state when continuous spatial symmetry is broken into discrete symmetry, so too should symmetry-breaking configure the spacetime crystal’s temporal component.
A spatial ring of trapped ions in persistent rotation would periodically reproduce itself in time, forming a temporal analog of an ordinary spatial crystal.
“While a space-time crystal looks like a perpetual motion machine and may seem implausible at first glance, keep in mind that a superconductor or even a normal metal ring can support persistent electron currents in its quantum ground state under the right conditions,” says Li.
It could help answer questions, such as how a space-time crystal emerges, how time translation symmetry breaks, and what are the quasi-particles in space-time crystals. It might also be used to store and transfer quantum information across different rotational states in both space and time.
“As there has never been a space-time crystal before, most of its properties will be unknown and we will have to study them,” says Xiang Zhang. “Such studies should deepen our understandings of phase transitions and symmetry-breaking.”