A team of Stanford University researchers has managed to create the world’s first complete computer model of an organism.
The team – led by Prof. Markus Covert – leveraged data from more than 900 scientific papers to account for every molecular interaction that takes place in the life cycle of Mycoplasma genitalium, the world’s smallest free-living bacterium.
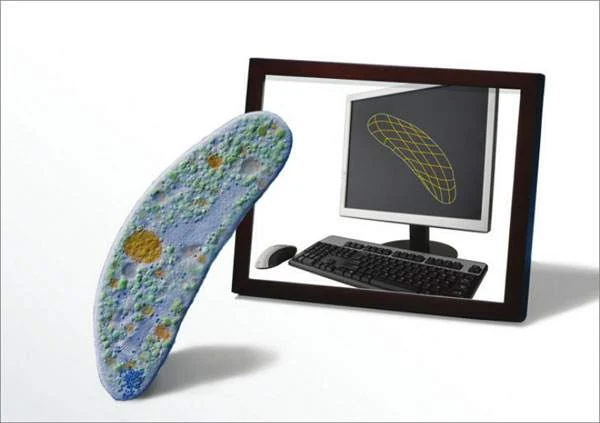
Not only does the model allow researchers to address questions that aren’t practical to examine otherwise, it represents a major stepping-stone toward the use of computer-aided design in bioengineering and medicine.
As Covert notes, recent developments in the field of biology has been marked by the rise of high-throughput studies producing staggering troves of cellular information. As such, a lack of experimental data is no longer the primary limiting factor for researchers. Instead, it’s how to make sense of what they already know.
However, most biological experiments, still adopt a reductionist approach to this vast array of data – knocking out a single gene and seeing what happens.
“[Yet], many of the issues we’re interested in aren’t single-gene problems,” Covert explained. “They’re the complex result of hundreds or thousands of genes interacting.”
According to Stanford bioengineering graduate student and co-first author Jayodita Sanghvi, this situation has resulted in a yawning gap between information and understanding that can only be addressed by “bringing all of that data into one place and seeing how it fits together.” Indeed, integrative computational models clarify data sets whose sheer size would otherwise place them outside human ken.
“You don’t really understand how something works until you can reproduce it yourself,” said Sanghvi.
Mycoplasma genitalium is a humble parasitic bacterium known mainly for arriving uninvited in human urogenital and respiratory tracts. But the pathogen also has the distinction of containing the smallest genome of any free-living organism – only 525 genes, as opposed to the 4,288 of E. coli, a more traditional laboratory bacterium.
Despite the difficulty of working with this sexually transmitted parasite, the minimalism of its genome has made it the focus of several recent bioengineering efforts. Notably, these include the J. Craig Venter Institute’s 2008 synthesis of the first artificial chromosome.
“The goal hasn’t only been to understand M. genitalium better,” said co-first author and Stanford biophysics graduate student Jonathan Karr. “It’s to understand biology generally.”
Even at this small scale, the quantity of data that the Stanford researchers incorporated into the virtual cell’s code was enormous – with the final model making use of more than 1,900 experimentally determined parameters.
To integrate these disparate data points into a unified machine, the researchers modeled individual biological processes as 28 separate “modules,” each governed by its own algorithm. These modules then communicated to each other after every time step, making for a unified whole that closely matched M. genitalium’s real-world behavior.
As noted above, the purely computational cell opens up procedures that would be difficult to perform in an actual organism, as well as opportunities to reexamine experimental data. A number of these approaches have already been demonstrated, including detailed investigations of DNA-binding protein dynamics and the identification of new gene functions.
The researchers had noticed, for example, that the length of individual stages in the cell cycle varied from cell to cell, while the length of the overall cycle was much more consistent. Consulting the model, the researchers hypothesized that the overall cell cycle’s lack of variation was the result of a built-in negative feedback mechanism.
Cells that took longer to begin DNA replication had time to amass a large pool of free nucleotides. The actual replication step, which uses these nucleotides to form new DNA strands, then passed relatively quickly. Cells that went through the initial step quicker, on the other hand, lacked a nucleotide surplus. Replication ended up slowing to the rate of nucleotide production.
While these types of conclusions remain hypotheses until they’re confirmed by real-world experiments, they do promise to accelerate the process of scientific inquiry.
“If you use a model to guide your experiments, you’re going to discover things faster. We’ve shown that time and time again,” Covert added.